8.5 Aerobic Metabolism: Krebs Cycle and The Electron Transport System
So far, we have discussed the two most immediate energy systems; the ATP–CP system and glycolysis. Although these systems are able to provide ATP very quickly, their major limitation is their relatively short duration of action. The Krebs cycle and the Electron Transport Chain (ETC), collectively referred to as aerobic metabolism, can provide ATP on a virtually limitless basis as long as fuel (carbohydrate, fat, or protein) and oxygen are available. As seen in Figure 8.11, the primary purpose of the Krebs cycle is to generate high energy molecules such as ATP, NADH, and FADH2.Earlier, we discussed the role of NAD+, the coenzyme form of the B vitamin niacin, in glycolysis. FAD, the coenzyme form of the B vitamin Riboflavin, has the same function in the Krebs cycle. NAD+ and FAD pick up the hydrogens that were stripped off in the Krebs cycle to form NADH and FADH2 . The hydrogens are then transported to the ETC, where they are used to make ATP (Figure 8.12). After delivering the hydrogens to the ETC, the NAD+ and FAD can then return to the Krebs cycle to pick up more hydrogens. Don’t get caught up in the details of all the chemical reactions going on here. Just focus on the big picture concepts.
Figure 8.11 Pyruvate to Acetyl CoA and Krebs Cycle
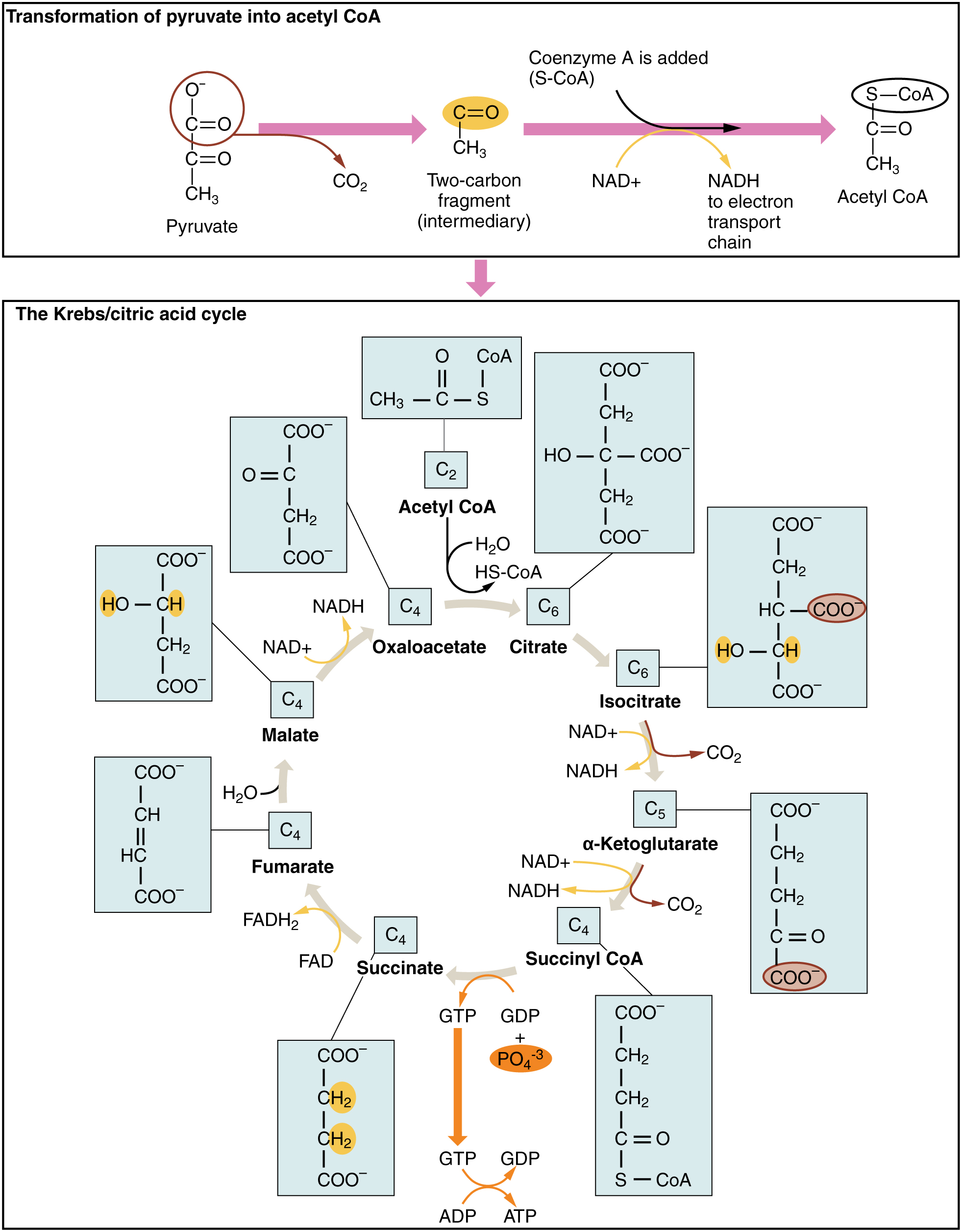
Figure 8.12 Electron Transport Chain
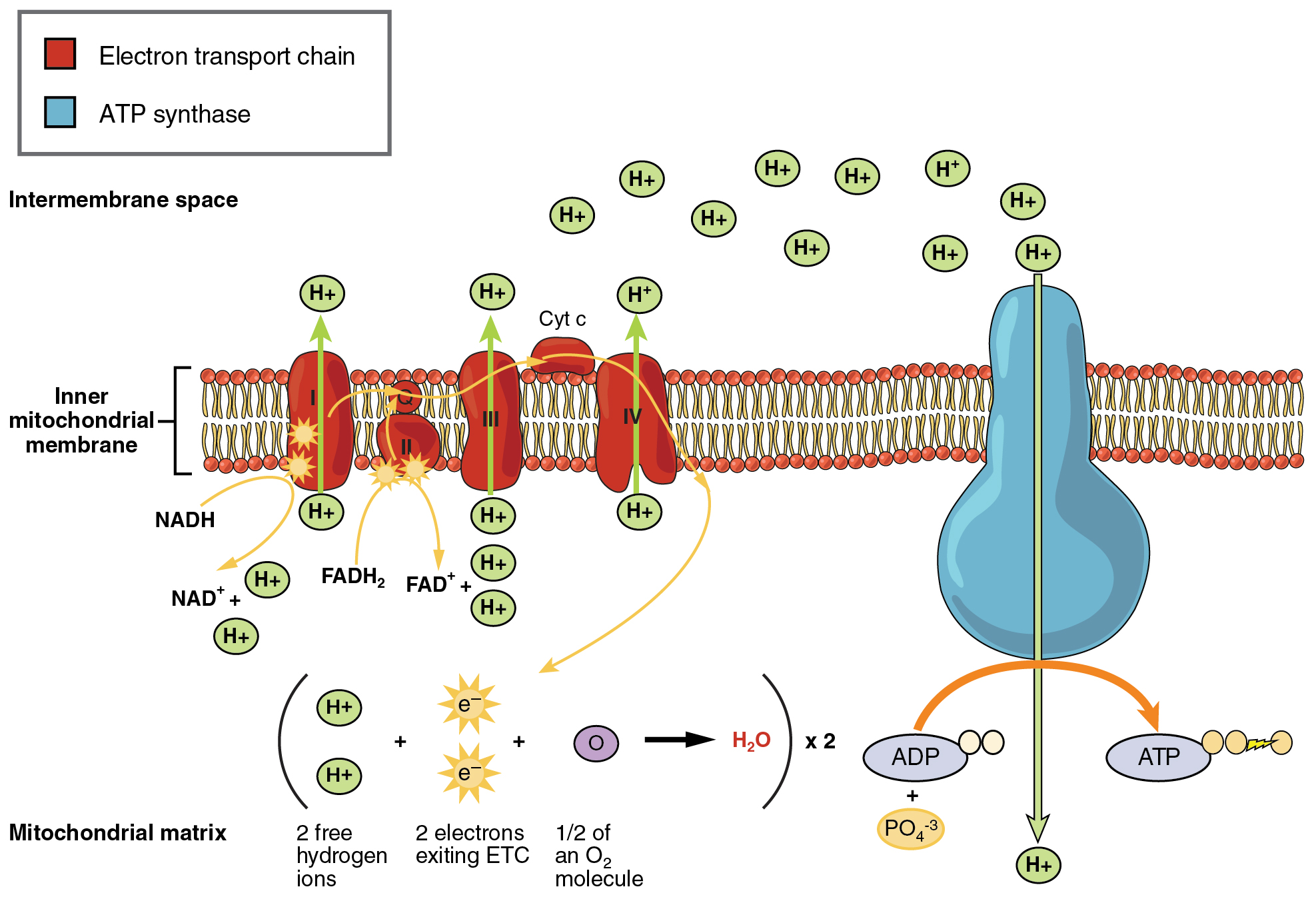
In aerobic metabolism, carbohydrate, protein, and fat can be used to generate ATP. This system is significantly more complex than anaerobic metabolism. If we follow glucose metabolism all the way through glycolysis and Krebs/ETC, we would find that it involves 124 steps. The ATP–CP system and glycolysis only involve 1 and 18 steps, respectively. Because of the complexity of aerobic metabolism, and the fact that it relies on oxygen delivery to the muscle cells (which takes time), it takes longer to “activate.” However, once it gets going, it can generate large amounts of ATP and support exercise for hours.
Let us start by looking at carbohydrate metabolism through Krebs/ETC. In order to make ATP from glucose in the aerobic system it must first go through glycolysis. Glycolysis ends with the production of two molecules of pyruvate. During anaerobic conditions (moderate-high to high-intensity exercise), most of the pyruvate is converted to lactate, but under aerobic conditions (low-to-moderate–intensity exercise) more is converted to a substance called acetyl CoA. The conversion of pyruvate to acetyl CoA (Figure 8.11) is the first point at which metabolism becomes oxygen dependent. In other words, we must have adequate amounts of oxygen in the muscle cells for it to occur. The shift from lactate to pyruvate production occurs gradually, as oxygen delivery increases in the cells. The first few minutes of any aerobic activity relies on glycolysis for ATP production because oxygen delivery takes time. Oxygen has to enter the lungs, then be transported from the lungs via the blood to the exercising muscles and into the cells. As the volume of oxygen gradually increases in the cells, the cells gradually shift from making lactate and ATP in glycolysis, to making acetyl CoA and the majority of the ATP in Krebs/ETC. We can also think of this as a shift from the anaerobic system to the aerobic system. Keep in mind, glycolysis never “shuts off” completely, it is still active but to a lesser degree. The acetyl CoA then enters the aerobic energy systems (Krebs/ETC) to make ATP. When all is said and done, one molecule of glucose can provide 30-33 ATP via aerobic metabolism. That is more than 10 times the number of ATP than what glucose yields via glycolysis alone.
Fats and proteins cannot enter glycolysis and therefore must go through other pathways in order to enter aerobic metabolism. Fatty acids from stored triglycerides can be used to create ATP via aerobic metabolism. Before they can be used for energy, triglycerides must be released from adipose tissue and broken down into glycerol and free fatty acids through a process called lipolysis. Once mobilized, the fatty acids are transported, bound to the blood protein albumin, to the cells of the body. After entering the muscle cell, they first have to go through a separate process called beta-oxidation, or fatty acid oxidation, before entering aerobic metabolism. During beta-oxidation, fatty acids composed of long chains of carbons are broken down into two carbon acetyl-CoA molecules. With each pass through beta oxidation, two carbons are removed from the end of the fatty acid chain. Each pass also results in the formation of one NADH and one FADH2. The two carbon fragments are converted to acetyl CoA which then enter aerobic metabolism to make ATP. Depending on the length of the fatty acids, one molecule of fat can provide hundreds of ATP (the longer the fatty acid, the more ATP produced)! However, as lipolysis and beta-oxidation are more complex than glycolysis, ATP production from fat is a much slower process than ATP production from carbohydrate. At rest, this is not important, but during high intensity exercise when ATP needs to be produced as quickly as possible, the working muscles will rely on carbohydrate as the primary energy source.
Amino acids from protein can also be used to make ATP in aerobic metabolism. However, it is important to note that protein is not a major storage form of energy like carbohydrate and fat. Most protein in the body is incorporated into tissues, such as lean muscle mass, and other substances such as enzymes. Of the three macronutrients, protein is the least preferred source of energy. There are certain circumstances where protein becomes a more significant source of energy production. Generally, protein becomes more important during metabolically stressful situations such as starvation and the later stages of prolonged exercise (the end of an iron man triathlon, marathon, or ultraendurance event). Under normal metabolic conditions, protein provides approximately 1%–2% of your overall energy production, but under stressful circumstances, it can provide more. Before protein can be used in aerobic metabolism, it must first be deaminated (removal of nitrogen). The nitrogen is then eliminated from the body in the urine as urea. Protein metabolism is complex, because there are 20 amino acids and several different entry points into aerobic metabolism. The number of ATP provided by an amino acid is variable, and is based on where that specific amino acid enters into metabolism. Regardless of the entry point, amino acids do not provide more than 15 ATP which is significantly less ATP than is provided by carbohydrate and fat. Furthermore, energy production from protein requires deamination and the production of urea, both of these processes use energy, which makes protein much less efficient. Another issue is that the use of protein generally comes at a cost. Most amino acids used for energy production are broken down out of muscle tissue, a condition that is generally not beneficial for an athlete or someone looking to lose or maintain weight in a healthy manner.
Aerobic metabolism is the predominant source of ATP production during endurance types of activities. Since oxygen delivery to the exercising muscles is the limiting factor for the use of these systems, aerobic metabolism requires approximately 1½ to 2 min to “take over” energy production. Once it kicks in, it can support ATP production for hours. The aerobic system is the primary producer of ATP for any activity lasting longer than 2 min up to hours of activity.
Media Attributions
- Krebs Cycle © OpenStax is licensed under a CC BY (Attribution) license
- Electron Transport System © OpenStax is licensed under a CC BY (Attribution) license