11 Neuromechanics of Cyclists
Guy Leshem
Chapter: Neuromechanics in Cycling
Sensory Feedback in Cyclists
Sensory feedback is a key part of how our nervous system works. It helps us feel andrespond to what’s happening inside our bodies and in the world around us. This includes things like proprioception (knowing where your body is in space), and tactile senses like pressure, vibration, and pain (Proske & Gandevia, 2012; Levine, 2007). These systems help us move smoothly, stay balanced, and react to our surroundings (Wolpert et al., 2011).
In cycling, there are only three contact points between the rider and the bike: the handlebars, saddle, and pedals. Many cyclists wear gloves for grip and comfort, but gloves can reduce sensitivity in the hands, which may affect how well a rider controls the bike.
More experienced riders often use clipless pedals. These connect their shoes directly to the pedals, which helps them pull up during each pedal stroke and not just push down. These pedals increase the amount of feedback the rider gets from the bike, making them feel more connected and reducing the need to think about foot position.
Terrain and Tactile Adjustment
When speed, terrain, or surface conditions change, riders adjust their grip and body position. They squeeze the bars tighter on rough roads or loosen their grip on smooth sections. Braking is another example—cyclists use feedback from the brake levers to apply the right amount of pressure and avoid skidding, especially in wet or loose conditions.
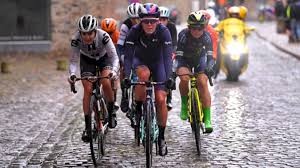
Mechanoreceptors in the arms help riders steer and make tiny corrections through the handlebars. Experienced cyclists can feel how their tires grip the road or trail and adjust their body to stay balanced, even in sketchy moments that could lead to a crash.
The muscle spindles and Golgi tendon organs help riders judge how much force their muscles are using. This is important when deciding whether to shift gears. If a rider is pedaling too slowly and using too much force, it makes sense to switch to an easier gear to avoid tiring the muscles too fast.
The Role of Wind in Road Cycling
For road cyclists, wind becomes a big part of sensory feedback. Knowing the direction and strength of the wind can help a rider conserve energy, gain speed, or even win a race. Cyclists use many cues to sense wind: they feel it on their face or skin, see flags or trees moving, and even notice how the bike leans in the wind.
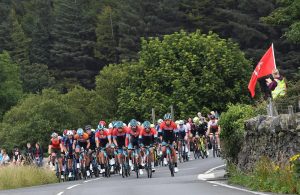
As speed increases, so does wind resistance. Cyclists in a group will try to “hide” from the wind by drafting behind others. Those who can’t avoid the wind will work much harder. Riders skilled at reading the wind can change their position in the group or adjust their body posture to be more aerodynamic and save energy by not having to resist the force of the wind.
How do you know a cyclist’s facing a headwind?
Don’t worry, they’ll tell you—every 30 seconds 🙂
Summary
Even though cycling doesn’t involve fine hand movements like playing an instrument, sensory feedback plays a big role in performance. Cyclists use their sense of touch, position, and environmental awareness to ride safely and efficiently. The connection between the rider, the bike, the ground, and the surroundings is essential for skill development and success—whether riding for fun, endurance, or racing.
Cortical Processing in Cyclists
Cortical processing is how the brain handles sensory information and turns it into action. Signals from all over the body go to the somatosensory cortex in the parietal lobe, where they are analyzed. From there, the brain uses the premotor cortex and motor cortex to decide what to do. The premotor area plans movement, and the motor cortex sends the final commands to the muscles. This system is what allows us to make voluntary movements, quick reflexes, and smooth, skilled actions (Latash & Singh, 2024).
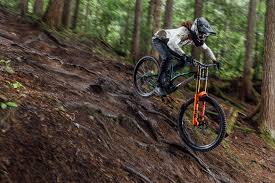
Quick Decisions in Real Life and Racing
In real-life cycling, fast decisions can be life-saving. A commuter might need to dodge a cat that runs into the bike lane. A pro cyclist might decide whether to sprint and chase a breakaway during a long stage race. A downhill racer, speeding down rocky trails, has to process trail information in split seconds to stay upright and fast.
This kind of fast thinking—taking in signals, processing them, and acting—is the heart of good cycling (Enders et al., 2016). That same research found that when cyclists get tired, the brain works even harder to keep everything under control.
Fatigue and Brain Activity
Studies have shown that during high-intensity or long rides, communication between brain areas increases (Brümmer et al., 2011). So even when the body is exhausted, the brain stays alert. This is crucial during the final parts of a race when tactics and smart choices—when to attack, what gear to choose, how hard to go—can decide the result.
But even top cyclists can make poor decisions under pressure. “Choking” is a real thing—stress and pressure can mess with how well the brain works leading to underperformance and disappointment (Smoulder et al., 2021). One reason may be dopamine levels: too little or too much can both hurt performance (Cools & D’Esposito, 2011).
In pro racing, where riders carry a team’s hopes and millions of fans are watching, staying calm and mentally sharp is not easy. Tools like EEG biofeedback may help, by giving riders feedback on their mental state and helping them improve focus by practicing how to control their mental state (Białkowska et al., 2019).
Summary
Cortical processing is critical in cycling. High speeds and changing conditions demand quick decisions. Riders must process sensory info fast and act effectively—even when tired or under pressure. Learning to manage fatigue and mental stress can make a difference between a good ride and a great one—or even between finishing a race and crashing out.
Motor Output in Cyclists
Motor output refers to the signals the brain sends to the muscles to make them move. In cycling, this is one of the most important parts of performance. The brain takes in info from the environment, processes it, and sends out commands to the muscles. At high speeds, decisions and actions need to be made quickly and accurately.
Reacting to Hazards
All cyclists—from beginners to pros—need fast reaction time to avoid crashes. Whether it’s a car door swinging open, a dog running across the street, or a teammate falling in front of you, the brain needs to recognize the danger and send the right message to the muscles right away.
Braking skill is key. Riders must know how much pressure to apply to each brake. Too much on the front brake can send you flying over the bars. With time and experience, riders learn how to control their brakes properly.
One study found that reaction time improves when exercise intensity goes up (Buchholtz & Burgess, n.d.). That suggests our brains become more alert during harder efforts.
Coordination and Pedaling Speed
Another important concept is the speed-accuracy trade-off. As movement speed goes up, accuracy can go down. In cycling, this applies more to coordination and power production. Some research shows that very high cadences (above 120–140 RPM) can reduce efficiency in some people due to poor coordination (Hodson-Tole et al., 2020).
Muscle Spindles and Golgi Tendons
Muscle spindles and Golgi tendon organs are the real heroes when thinking about muscle activation but are often underrated, they are special sensors in the muscles and tendons. Spindles detect changes in muscle length, helping with balance and timing. Golgi tendons measure tension. When it’s too high, they tell the brain to stop the muscle from over-contracting, protecting the body from injury, you can read more about motor output in chapter (Motoki, 2022).
In cycling, these sensors give important info about how much force to apply, when to push, and how to coordinate muscles efficiently to produce smooth and powerful pedal strokes (Kistemaker et al., 2013).
When your legs say “no,” but your brain says “go,”
That’s called Type II fun neuroscience.
Summary
Motor output is critical to cycling. From reacting to sudden dangers to producing smooth, efficient power, the brain and muscles must communicate quickly and clearly. Coordination, timing, and proper force application all rely on this system—and elite cyclists have spent years training it to work at the highest level.
Citation:
Białkowska, J., Mroczkowska, D., & Wickland-Białkowska, M. (2019). The use of EEG biofeedback to improve memory, concentration, attention and reduce emotional tension. Rehabilitacja Medyczna, 23(2), 31–35. https://doi.org/10.5604/01.3001.0013.5097
Brümmer, V., Schneider, S., Strüder, H. K., & Askew, C. D. (2011). Primary motor cortex activity is elevated with incremental exercise intensity. Neuroscience, 181, 150–162. https://doi.org/10.1016/j.neuroscience.2011.02.006
Buchholtz, K., & Burgess, T. L. (n.d.). An evaluation of bicycle-specific agility and reaction times in mountain bikers and road cyclists. South African Journal of Sports Medicine, 32(1), v32i1a8576. https://doi.org/10.17159/2078-516X/2020/v32i1a8576
Cools, R., & D’Esposito, M. (2011). Inverted-U–Shaped Dopamine Actions on Human Working Memory and Cognitive Control. Biological Psychiatry, 69(12), e113–e125. https://doi.org/10.1016/j.biopsych.2011.03.028
Enders, H., Cortese, F., Maurer, C., Baltich, J., Protzner, A. B., & Nigg, B. M. (2016). Changes in cortical activity measured with EEG during a high-intensity cycling exercise. Journal of Neurophysiology, 115(1), 379–388. https://doi.org/10.1152/jn.00497.2015
HODSON-TOLE, E. F., BLAKE, O. M., & WAKELING, J. M. (2020). During Cycling What Limits Maximum Mechanical Power Output at Cadences above 120 rpm? Medicine and Science in Sports and Exercise, 52(1), 214–224. https://doi.org/10.1249/MSS.0000000000002096
Kistemaker, D. A., Van Soest, A. J. K., Wong, J. D., Kurtzer, I., & Gribble, P. L. (2013). Control of position and movement is simplified by combined muscle spindle and Golgi tendon organ feedback. Journal of Neurophysiology, 109(4), 1126–1139. https://doi.org/10.1152/jn.00751.2012
Latash, M. L., & Singh, T. (2024). Neurophysiological basis of Motor Control Third Edition. Human Kinetics.
Levine, D. N. (2007). Sherrington’s “The Integrative action of the nervous system”: A centennial appraisal. Journal of the Neurological Sciences, 253(1–2), 1–6. https://doi.org/10.1016/j.jns.2006.12.002
Motoki. (n.d.). Motor Output. Retrieved April 15, 2025, from https://pressbooks.calstate.edu/humanneuromechanics/chapter/motor-output/
Proske, U., & Gandevia, S. C. (2012). The proprioceptive senses: Their roles in signaling body shape, body position and movement, and muscle force. Physiological Reviews, 92(4), 1651–1697. https://doi.org/10.1152/physrev.00048.2011
Smoulder, A. L., Pavlovsky, N. P., Marino, P. J., Degenhart, A. D., McClain, N. T., Batista, A. P., & Chase, S. M. (2021). Monkeys exhibit a paradoxical decrease in performance in high-stakes scenarios. Proceedings of the National Academy of Sciences, 118(35), e2109643118. https://doi.org/10.1073/pnas.2109643118
Wolpert, D. M., Diedrichsen, J., & Flanagan, J. R. (2011). Principles of sensorimotor learning. Nature Reviews. Neuroscience, 12(12), 739–751. https://doi.org/10.1038/nrn3112
visual, vestibular, or somatosensory feedback
The sense or perception of the position, movement, and spatial orientation of one's body and its limbs
Sensory receptors that respond to mechanical pressure or distortion. They are in the skin, muscles, internal organs, and other tissues, and they help detect sensations such as touch, pressure, vibration, stretch, and sound.
Specialized sensory receptor found within skeletal muscles that is responsible for monitoring changes in muscle length and providing proprioceptive feedback to the central nervous system
Sensory receptor found in tendons, which are the tough bands of connective tissue that attach muscles to bones.
somatosense related to position and touch as well as sense of vibration, pain, temperature relayed to somatosensory areas of the parietal cortex
A sudden and significant decline in performance in high-pressure situations